Polar Vs. Nonpolar Molecules: Explained + Examples | Chemistry
Ever wondered why oil and water don't mix, or how certain substances dissolve while others remain stubbornly separate? The answer lies in the fascinating world of molecular polarity, a fundamental concept that governs how molecules interact and behave.
This article delves into the core principles of polarity, exploring its nuances and implications. We'll uncover the forces that drive these molecular behaviors, revealing why some substances embrace interaction while others remain aloof.
At its heart, polarity concerns the distribution of electrical charge within a molecule. Some molecules, like water (HO), exhibit an uneven distribution of electrons, creating regions of partial positive and partial negative charge. These are known as polar molecules. Others, like methane (CH), share electrons more equally, resulting in a more neutral distribution of charge and are termed nonpolar. The difference in electronegativity, the ability of an atom to attract electrons, between the atoms involved in a bond is the key factor that determines the polarity of a molecule.
Let's take a look at the basic characteristics of polar and nonpolar molecules through a table:
Property | Polar Molecules | Nonpolar Molecules |
---|---|---|
Charge Distribution | Uneven, creating a dipole moment (a separation of charge) | Even, with no significant dipole moment |
Electronegativity Difference | Significant difference between bonded atoms (typically >0.4) | Small or no difference between bonded atoms (typically <0.4) |
Examples of Polar molecules | Water (HO), Ammonia (NH), Ethanol (CHOH) | Methane (CH), Carbon Dioxide (CO), Benzene (CH) |
Solubility | Dissolve in polar solvents (e.g., water) | Dissolve in nonpolar solvents (e.g., hexane) |
Intermolecular Forces | Dipole-dipole interactions, hydrogen bonding (in some cases) | London dispersion forces (weakest type of intermolecular force) |
Melting and Boiling Points | Generally higher than nonpolar molecules of similar size | Generally lower than polar molecules of similar size |
Surface Tension | Generally higher than nonpolar molecules of similar size | Generally lower than polar molecules of similar size |
Vapor Pressure | Generally lower than nonpolar molecules of similar size | Generally higher than polar molecules of similar size |
For more detailed information, you can refer to the following website: https://chem.libretexts.org/Bookshelves/Introductory_Chemistry/Book%3A_Introductory_Chemistry_(CK-12)/05%3A_Chemical_Bonds_and_Molecular_Geometry/5.07%3A_Polarity_of_Molecules
The foundation of polarity lies in the types of bonds that hold atoms together. A nonpolar bond forms when two nonmetals share electrons equally. This typically occurs between atoms of the same element or between atoms with very similar electronegativity values. The result is a bond with no significant charge separation.
Conversely, a polar bond forms when electrons are shared unequally. This happens when atoms of different elements with different electronegativity values combine. The more electronegative atom attracts the shared electrons more strongly, creating a partial negative charge (-) on that atom and a partial positive charge (+) on the other atom. The difference in electronegativity is a reliable measure of the bond's polarity: a difference of less than approximately 0.4 typically indicates a nonpolar bond, while a difference greater than 0.4 suggests a polar bond.
It is crucial to distinguish between polar bonds and polar molecules. While a molecule must contain polar bonds to be polar, it's not always a guarantee. Molecular geometry also plays a critical role. For a molecule to be polar, it needs to exhibit a certain level of asymmetry. Consider carbon dioxide (CO), a molecule with two polar C=O bonds. However, the linear shape of the molecule ensures the dipoles cancel each other out, making CO nonpolar. In contrast, water (HO) has a bent shape, and its polar O-H bonds do not cancel each other, resulting in a net dipole moment and making water a polar molecule.
The "like dissolves like" principle is a crucial concept. Polar solvents, like water, readily dissolve polar solutes because the attractive forces between the solvent and solute molecules are strong. Similarly, nonpolar solvents dissolve nonpolar solutes. This principle underpins many phenomena, from simple mixing to complex chemical reactions. For example, ionic compounds, the ultimate example of polar bonds, are generally soluble in polar solvents. Nonpolar molecules, on the other hand, are less soluble in polar solvents due to the weaker interactions between them.
A diatomic molecule formed by a polar covalent bond, such as hydrogen fluoride (\(\ce{HF}\)), provides a clear example of a polar molecule. Because the fluorine atom is more electronegative than the hydrogen atom, the shared electrons are pulled closer to the fluorine, creating a dipole moment within the molecule.
Understanding polarity provides valuable insights into the characteristics and behaviors of compounds. Polar molecules are frequently associated with higher melting and boiling points, surface tension, and lower vapor pressure than nonpolar molecules of comparable size. These differences stem from the stronger intermolecular forces present in polar substances. Polar molecules can engage in dipole-dipole interactions, which are the attractive forces between the positive and negative ends of polar molecules. Hydrogen bonding, a particularly strong form of dipole-dipole interaction, can occur between molecules containing hydrogen bonded to highly electronegative atoms like oxygen, nitrogen, or fluorine. Nonpolar molecules, lacking a permanent dipole moment, mainly rely on weaker London dispersion forces, which arise from temporary fluctuations in electron distribution.
The concept of polarity also has significant implications in cell biology. For instance, the way that molecules interact with water is a fundamental aspect of how life functions. Polar amino acids, with their ability to form hydrogen bonds and interact with water, are indispensable for the structure and function of proteins. They contribute to protein stability and enable crucial biological processes. Water, a polar molecule, can interact with other polar molecules and ionic compounds.
Polarity extends beyond the basic understanding of solubility and reactivity; it shapes the interactions within complex systems. In custom formulations, such as those involving oils or chemical blends, knowing the polarity of each component is crucial. Polar solvents like water interact effectively with polar solutes, which allows for tailored combinations based on these properties. Nonpolar molecules might not interact as effectively with polar solvents and could form separate layers, depending on the formulation and the solvent being used. The choice of solvent and solute hinges on these distinctions.
Consider the structure and geometry of molecules. For a molecule to be polar, it must possess polar covalent bonds and exhibit geometric asymmetry. The arrangement of the bonds around the central atom directly impacts a molecule's overall polarity. Even if a molecule contains polar bonds, its overall polarity may be non-existent if those bonds are symmetrically arranged. Symmetrical nonpolar molecules include carbon dioxide (CO2), which has two polar C=O bonds but is linear, and is therefore nonpolar, because the dipole moments cancel each other out.
Nonpolar molecules, which either do not have polar bonds or have symmetrical arrangements of polar bonds, typically exhibit different characteristics. They often show weaker interactions, which affects their behavior within solvents, and potentially limits their reactivity. Boron trifluoride (BF3) is a prime example of a nonpolar molecule due to its trigonal planar geometry and the equal distribution of three identical peripheral atoms.
The degree of polarity within a compound can vary considerably. Some bonds between different elements are only minimally polar, while others are highly polar. Ionic bonds are considered the ultimate in polarity, where electrons are transferred instead of shared.
In conclusion, the concept of polarity serves as a crucial tool for understanding and predicting molecular behavior. Whether predicting solubility, interpreting chemical reactions, or appreciating the intricacies of biological systems, a firm grasp of polarity unlocks a deeper comprehension of the chemical world.


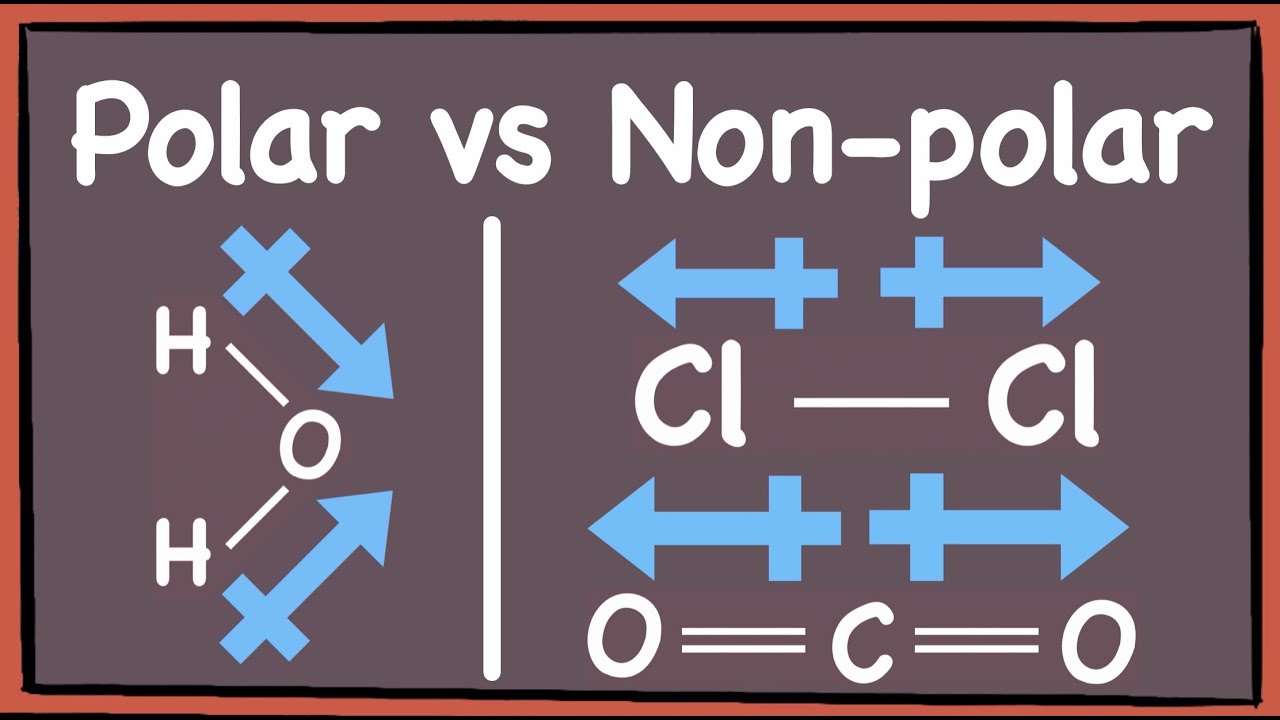